Long-term effects of recurrent seizures on
learning, behavior and anxiety: an
experimental study in rats
Xiu-Yu Shi, Ji-Wen Wang, Ge-Fei Lei, Ruo-Peng Sun
Jinan, China
Author Affiliations: Department of Pediatrics, Qilu Hospital, Shandong University, Jinan 250012, China (Shi XY, Wang JW, Lei GF, Sun RP)
Corresponding Author: Ruo-Peng Sun, MD, Department of Pediatrics, Qilu Hospital, Shandong University, No. 107 Wen Hua Xi Road, Jinan 250012, China (Tel: 86-13854187216; Fax: 86-531-86927544; Email: sunruopeng1948@yahoo.com.cn)
Background: Animal models have suggested that seizures in the developing brain cause less macroscopic structural damage than seizures in adulthood but accumulating evidence shows that recurrent seizures in infants are associated with a high incidence of cognitive deficits. In this study, we determined the effects of recurrent neonatal seizures on cognitive tasks using a set of behavioral paradigms when the animals were in adulthood.
Methods: Recurrent seizures were induced by injecting rat pups with pilocarpine on postnatal day 2 (P2), P4 and P7. In adulthood (P52-P56), spatial learning was examined by the Morris water maze; activity level was assessed by an open field test; and anxiety was examined by the elevated plus maze.
Results: Compared with controls, rats with recurrent seizures had deficient spatial learning and a greater degree of anxiety.
Conclusions: Our findings indicate that recurrent seizures during the neonatal period result in life-long impairment of certain cognition.
Key words: epilepsy; development; pilocarpine; long-term effects; behavioral testing; water maze
World J Pediatr 2007;3(1):61-65
Introduction
Seizures occur more frequently in the neonatal period than at any other time in life. In addition to the increased risk for epilepsy in children, seizures during early development may be more detrimental than those during adulthood.[1] Clinical studies suggest that seizures during development can produce adverse long-term consequences. The mean IQ score in children with seizures is lower than that in the normal population.[2,3] In addition to problems with memory and other cognitive processes, many patients with epilepsy have also been diagnosed with affective and personality disorders. However, there is ongoing clinical discussion of the possible damage induced by epileptic activity in the brains of infants and children.[4,5] Otherwise, the effects of recurrent seizures in immature animals were also controversial. For example, Sarkisian et al[6] reported that immature rats were spared from long-term cognitive and pathologic sequelae after repeated doses of kainate-induced status epileptics (SE). But other experiments[7-9] demonstrated that electrically or chemically (e.g., kainic acid, pilocarpine, lithium-pilocarpine) induced seizures in rat pups resulted in significant cognitive deficits and pathologic changes. In those experiments, the most commonly tested functions were spatial memory and learning functions,[10,11] but there have been few studies to date which examined the effects of recurrent seizures on emotionality in immature rats. Therefore the aim of the present study was to investigate the effects of pilocarpine-induced recurrent seizures on learning, behavior and anxiety in immature rats over a long period in this follow-up study.
Methods
Animals
Neonatal Wistar rats were obtained from the Experimental Animal Center of Shandong University. The birth date was designated as postnatal day 0 (P0). The animals had access to food and water ad libitum and were housed with their littermates till weaning on P21, when they were group-housed in plastic cages on a standard 12-hour light/dark cycle. Experimental or control animals remained together with their mother until weaning.
Induction of seizure
Pilocarpine (Sigma, St, Louis, MO, USA) was dissolved in distilled water at a concentration of 100 mg/ml and administered intraperitoneally (i.p.) to experimental neonatal rats on P2, P4 and P7 at a dosage of 350 mg/kg. All animals received scopolamine (1 mg/kg, i.p.) 30 minutes before pilocarpine administration to limit peripheral cholinergic effects. The animals were monitored throughout seizure induction, and seizure severity was assessed according to the scale of Lado.[12] The behavioral seizure scale in rat pups was summarized by Lado as follows: stage 0, behavioral arrest; stage 1, mouth clonus; stage 2, head bobbing; stage 3, unilateral forelimb clonus; stage 3.5, alternating forelimb clonus; stage 4, bilateral forelimb clonus with rearing; stage 5, bilateral forelimb clonus with rearing and falling over; stage 6, wild running and jumping with vocalization; and stage 7, tonus. If the animals did not show obvious signs of seizure 30 minutes after pilocarpine administration, additional dosage of 10 mg/kg pilocarpine was given every 10 minutes until the onset of seizure. If the convulsions lasted more than 30 minutes, chloral hydrate (400 mg/kg) was administered to the rats to terminate seizures. Control rats were given an equal volume of saline.
Experimental procedures
Thirty rats [experimental (exp), n=18; control (con), n=12] were used in this study. Two rats of the experimental group died after recurrent seizures, 12 rats were chosen from the live experimental group for further study. None of the control group died. Experimental and control rats were stochastically divided into two groups: group I and group II, 12 rats in each group (exp, n=6; con, n=6). Group I was tested for spatial memory by Morris water maze on P52. Group II was tested by the open-field test and elevated plus maze since P52.
Morris water maze
On P52, rats of group I were tested with water maze for 4 days. A stainless-steel circular swimming pool (117 cm in diameter, 50 cm high) was filled to a depth of 25 cm with water. Two hundred milliliters of evaporated milk was added to make the water opaque and prevent visualization of the platform. Four points on the rim of the pool were designated north (N), south (S), east (E), and west (W), thus dividing the pool into four quadrants (NW, NE, SE, SW). An 8 ¡Á 8 cm plexiglass platform, onto which the rat could escape, was positioned in the center of one of the quadrants, 1 cm below the water surface. One day before the test, each rat was placed in the pool for 60 seconds without the platform present; this free swim enabled the rat to become habituated to the training environment. On day 1 through 4, the rat was trained for 24 trials (6 trials per day) to locate and escape onto the submerged platform. For each rat, the quadrant in which the platform was located remained constant, but the point of immersion into the pool varied between N, E, S, and W in a quasi-random order for the 24 trials, so that the rat was not able to predict the platform location from the point at which it was placed into the pool. The latency from immersion into the pool to escape onto the platform was recorded for each trial. If the rat did not find the platform in 120 seconds it was manually placed on the platform for a 30-second rest. On day 5, the platform was removed. The rat was allowed 60 seconds of free swimming. The time spent in the quadrant where the platform was previously located was measured (probe trial), which was considered to assess memory for platform location.
Open-field test
On P52, rats of group II were tested in the open-field test. The floor of a square plastic board (50 ¡Á 50 cm) with plastic sides (30 cm high) was divided into 16 squares. The rats were individually placed in one corner of the open field and allowed to explore the area freely. The activity level was expressed as the total number of squares crossed, whereas exploratory activity was expressed as the total number of rearings during a 5-minute testing period. The open-field apparatus was cleaned using alcohol before the next animal is introduced, to preclude the possible cuing effects of odors left by previous subjects.[13]
Elevated plus maze
After the open-field test, rats of group II were tested in the elevated plus maze. The elevated plus-maze apparatus was made of wood-block and consisted of four arms (39.5 cm long ¡Á 10 cm wide) fixed to a central platform (10 cm ¡Á 10 cm): two had 12 cm high walls (closed-arms) and two had no border in place of the walls (open-arms). The maze was elevated to a height of 40 cm. The rat was brought individually to the testing room 1 hour before the test was started. For testing, the rat was placed in the center of the maze, its head facing an open-arm (the same for all mice) and its behavior was recorded by a group of experimenters, unaware of the treatments, for a period of 5 minutes. The primary measurements were the number of entries and the time spent in each arm. For the evaluation of anxiety related animal behavior, the percentage of time spent on open/total arms and the percentage of open/total arm entries [open/(close + open) ¡Á 100] were measured as conventional anxiety indices.
Statistical analysis
All results were shown as mean¡ÀSEM. Differences between means of the two groups were compared using Student's t test or the Mann-Whitney u test, depending on whether the data were normally distributed. SPSS for Windows 11.5 software was used for statistical analysis. In all cases, significance level was set at P<0.05.
Results
Morris water maze
All rats learned to find platform and escape onto it, as indicated by the decrease in mean escape latency over the 4 training days in each group. The rats with recurrent seizures were significantly slower in finding the platform than the controls (Table). It was noteworthy that the differences of mean escape latencies in pilocarpine-treated rats were most significant on the first (P<0.001) and second days (P<0.001). When the probe trial was tested, both groups of rats spent more time in the goal quadrant. But there was a significant difference in time spent in the goal quadrant between the two groups (Mann-Whitney u test=5.5, P=0.041; Fig.).
Table. Mean latencies across trials in the Morris water maze task (mean¡ÀSEM)
Group (n)
|
1 d
|
2 d
|
3 d
|
4 d
|
Experimental (6)
|
57.6¡À4.3 s
|
40.3¡À2.5 s
|
29.6¡À2.9 s
|
26.3¡À2.9 s
|
Control (6)
|
41.9¡À2.7 s
|
29.9¡À4.1 s
|
24.7¡À2.0 s
|
22.26¡À2.2 s
|
t
|
7.625
|
5.365
|
3.35
|
2.765
|
P
|
<0.001
|
<0.001
|
0.007
|
0.002
|
Comparison of Morris water maze performance in control rats and experimental rats after recurrent seizures. There were differences in performance on all testing days (P<0.05).
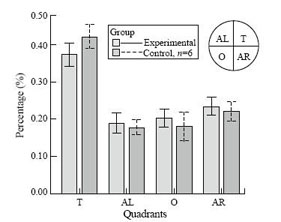
Fig. The effects of pilocarpine-induced seizures on the probe trials of Morris water maze performance. Graphs showing the mean percentage of total time swimming in each quadrant for control and experimental rats.
Open-field test
We measured the total number of blocks and number of rears in a 5-minute period. There was no significant difference in either number of blocks crossed (activity level, Mann-Whitney u test =10.0, P>0.05) or number of rearings (exploratory activity, Mann-Whitney u test=13.0, P>0.05).
Elevated plus maze
A significant decrease in the percentage of time spent in open-arms (Mann-Whitney u test <0.01, P<0.01) and in the percentage of entries to open-arms (Mann-Whitney u test =2.5, P<0.05) was observed in the rats with recurrent seizures. These findings demonstrated that increased anxiety existed in pilocarpine-treated rats.
Discussion
A number of previous studies evaluating the effects of seizures on the developing brain have suggested that the immature brain is less susceptible to seizure-induced brain damage than the mature brain.[14-16] However, this decreased vulnerability is not absolute since more and more experiments[7-9,17] demonstrated that electrically or chemically induced seizures in rat pups resulted in significant cognitive deficits, alterations in seizure susceptibility and pathologic changes. This study systematically evaluated the effects of recurrent seizures on learning, behavior and anxiety when the rats matured. The principal findings were that rats with recurrent seizures had deficient spatial learning and greater degree of anxiety. These results add to the accumulating evidence that seizure during early brain development can result in life-long behavioral and cognitive deficits.
In this study, we used a measure of hippocampal integrity, the water maze, a well-validated test of spatial learning and memory. In this task, the rats that were treated with pilocarpine learned the platform location slower than the controls. On the probe component of the water maze, the rats were allowed to swim freely without a platform present, to assess their preference (memory) for platform location in the target quadrant. Pilocarpine treated group spent significantly less time swimming in the target quadrant than controls, suggesting that the experimental group had poorer memory of platform location. Although several factors may account for spatial learning deficits, a likely explanation may be the mossy fiber sprouting. There are now considerable indirect evidence that these new neurites establish functional synaptic connections[18,19] and the degree of stratum pyramidale mossy fiber projection correlates with learning. Lipp et al[20] showed that the magnitude of the stratum pyramidale projections of mossy fibers correlated with the number of trials to criterion in two-way avoidance learning with animals having more CA3 mossy fiber terminals doing less well than animals with fewer terminals. Crusio et al[21] found that the size of the hippocampal stratum pyramidale and infrapyramidal mossy fiber terminal field correlated inversely with the error number in a radial maze test. This study appears to provide a structural substrate for augmentation of synaptic activity that is worthy of further study.
Furthermore, we used an open-field test to evaluate behavioral changes of rats. In open-field test, no difference was seen in locomotor activity or exploratory activity between the control and experimental rats. The case is not the same in adult rats; it has been demonstrated that hippocampal neuronal loss and open-field motor activity decreased after pentylenetetrazole kindling in mature rats over long periods.[22] But in genetically epilepsy-prone rats, frequent and brief seizures caused less motor activity in the open-field test.[23] All these studies have shown that behavioral changes are related to the age of rats and type of seizure.
We subjected all rats to the elevated plus maze to evaluate emotional memory and learning performance. Indeed, the elevated plus-maze is one of the most popular models currently used in the study of animal anxiety.[24] In this test, the rats that experienced recurrent seizures at these early ages exhibited heightened levels of anxiety as adults, again correlating with abnormal hippocampal/limbic system function. These findings correspond to the clinical report that many patients with epilepsy have also been diagnosed with affective and personality disorders.[25] We have described that there was no difference in open-field test, therefore the difference in anxiety scores between control and experimental rats was not due to an alteration of locomotor or exploratory activity and may therefore be a consequence associated with the seizure history. But how seizures result in anxiety-related behavior changes remains unclear. It is generally accepted that antenatal and/or early postnatal stress adversely affects human development, increasing the susceptibility to diseases later in life,[26] as well as altering behavioral and cognitive development.[27] These effects have been linked to persistent alterations in the hormonal and metabolic responses to stress associated with changes in the regulation of the hypothalamic-pituitary-adrenal (HPA) axis. Neonatal rats experienced recurrent seizures in this study. Every seizure can be seen as a stress event, for previous study[28] has proved that plasma glucocorticosteroid level sustained increase following perinatal recurrent seizures. A large body of literature suggests that excess corticosterone is detrimental to hippocampal functions.[29,30] Recently, it has been confirmed that high levels of glucocorticoid (CORT) are thought to regulate all stages of neurogenesis.[31] New neurons in the hippocampus are responsible for the formation of new memory,[32] the detrimental effect of CORT on neurogenesis may account for the behavioral deficits which were observed in this study. Furthermore, many researchers[33,34] observed that raised levels of CORT can induce atrophy of the apical dendrites of hippocampal CA3 pyramidal neurons that appeared to retract their dendrites, which might have an impact on the total number of dendritic synapses. However, synapses also play an important role in hippocampus-dependent learning and memory, so the impairment of synapses induced by raised levels of CORT may be another cause of behavioral deficits.
In conclusion, on multiple measures of hippocampus-based cognitive functions, the rats that experienced recurrent seizures during early development had persistent deficits: in water maze acquisition learning and spatial memory as adults; and increased anxiety in elevated plus maze. These results suggest that seizures disrupt some aspect of hippocampal function during the early, vulnerable "critical period" of brain development, with deficits observable long afterward. These observations may have clinical implications for cognitive and memory dysfunction associated with epilepsy during development. Therefore, further investigation will involve gathering more information about clinical experiment and how to diminish the adverse effects of early perinatal seizures on learning, memory and anxiety.
Funding: This study was supported by a grant from Science and Technology Bureau of Shandong Province (No. 2004BS02015).
Ethical approval: Not needed.
Competing interest: None declared.
Contributors: SXY wrote the first draft of this paper. All authors contributed to the intellectual content and approved the final version.
Acknowledgements
The authors wish to thank Liu Chunxi, Liu Ping for their technical assistance with data collection and care of the animals during the study as well as Wang Yanling for editorial assistance.
References
1 Painter MJ, Bergman I, Crumrine P. Neonatal seizures. Pediatr Clin North Am 1986;33:91-109.
2 Farwell JR, Dodrill CB, Batzel LW. Neuropsychological abilities of children with epilepsy. Epilepsia 1985;26:395-400.
3 Huttenlocher PR, Hapke RJ. A follow-up study of intractable seizures in childhood. Ann Neurol 1990;28:699-705.
4 Holmes GL. Epilepsy in the developing brain: lessons from the laboratory and clinic. Epilepsia 1997;38:12-30.
5 Suchomelova L, Kubova H, Haugvicova R, Druga R, Mares P. Are acute changes after status epilepticus in immature rats persistent? Physiol Res 2002;51:185-192.
6 Sarkisian MR, Tandon P, Liu Z, Yang Y, Hori A, Holmes GL, et al. Multiple kainic acid seizures in the immature and adult brain: ictal manifestations and long-term effects on learning and memory. Epilepsia 1997;38:1157-1166.
7 Holmes GL, Gairsa JL, Chevassus-Au-Louis N, Ben-Ari Y. Consequences of neonatal seizures in the rat: morphological and behavioral effects. Ann Neurol 1998;44:845-857.
8 Majak K, Pitkanen A. Do seizures cause irreversible cognitive damage? Evidence from animal studies. Epilepsy Behav 2004;5:35-44.
9 Sayin U, Sutula TP, Stafstrom CE. Seizures in the developing brain cause adverse long-term effects on spatial learning and anxiety. Epilepsia 2004;45:1539-1548.
10 Wu CL, Huang LT, Liou CW, Wang TJ, Tung YR, Hsu HY, et al. Lithium-pilocarpine-induced status epilepticus in immature rats result in long-term deficits in spatial learning and hippocampal cell loss. Neurosci Lett 2001;312:113-117.
11 Rutten A, Van Albada M, Silveira DC, Cha BH, Liu X, Hu YN, et al. Memory impairment following status epilepticus in immature rats: time-course and environmental effects. Eur J Neurosci 2002;16:501-513.
12 Lado FA, Sperber EF, Moshe SL. Anticonvulsant efficacy of gabapentin on kindling in the immature brain. Epilepsia 2001;42:458-463.
13 Harro J, Haidkind R, Harro M, Modiri AR, Gillberg PG, Pahkla R, et al. Chronic mild unpredictable stress after noradrenergic denervation: attenuation of behavioural and biochemical effects of DSP-4 treatment. Eur Neuropsychopharmacol 1999;10:5-16.
14 Hirsch E, Baram TZ, Snead OC 3rd. Ontogenic study of lithium-pilocarpine-induced status epilepticus in rats. Brain Res 1992;583:120-126.
15 Stafstrom CE, Thompson JL, Holmes GL. Kainic acid seizures in the developing brain: status epilepticus and spontaneous recurrent seizures. Brain Res Dev Brain Res 1992;65:227-236.
16 Thurber S, Mikati MA, Stafstrom CE, Jensen FE, Holmes GL. Quisqualic acid-induced seizures during development: a behavioral and EEG study. Epilepsia 1994;35:868-875.
17 Kornblum HI, Sankar R, Shin DH, Wasterlain CG, Gall CM. Induction of brain derived neurotrophic factor mRNA by seizures in neonatal and juvenile rat brain. Brain Res Mol Brain Res 1997;44:219-228.
18 Okazaki ME, Evenson DA, Nadler JV. Hippocampal mossy fiber sprouting and synapse formation after status epilepticus in rats: visualization after retrograde transport of biocytin. J Comp Neurol 1995;352:515-534.
19 Wuarin JP, Dudek FE. Electrographic seizures and new recurrent excitatory circuits in the dentate gyrus of hippocampal slices from kainate-treated epileptic rats. J Neurosci 1996;16:4438-4448.
20 Lipp HP, Schwegler H, Heimrich B, Driscoll P. Infrapyramidal mossy fibers and two-way avoidance learning: developmental modification of hippocampal circuitry and adult behavior of rats and mice. J Neurosci 1988;8:1905-1921.
21 Crusio WE, Schwegler H, Lipp HP. Radial-maze performance and structural variation of the hippocampus in mice: a correlation with mossy fibre distribution. Brain Res 1987;425:182-185.
22 Franke H, Kittner H. Morphological alterations of neurons and astrocytes and changes in emotional behavior in pentylenetetrazol-kindled rats. Pharmacol Biochem Behav 2001;70:291-303.
23 Holmes GL, Thompson JL, Marchi TA, Gabriel PS, Hogan MA, Carl FG, et al. Effects of seizures on learning, memory, and behavior in the genetically epilepsy-prone rat. Ann Neurol 1990;27:24-32.
24 Bertoglio LJ, Carobrez AP. Prior maze experience required to alter midazolam effects in rats submitted to the elevated plus-maze. Pharmacol Biochem Behav 2002;72:449-455.
25 Dodrill CB. Neuropsychological effects of seizures. Epilepsy Behav 2004;5(Suppl 1):S21-24.
26 Barker DJ. Fetal programming of coronary heart disease. Trends Endocrinol Metab 2002;13:364-368.
27 Charmandari E, Kino T, Souvatzoglou E, Chrousos GP. Pediatric stress: hormonal mediators and human development. Horm Res 2003;59:161-179.
28 Liu H, Kaur J, Dashtipour K, Kinyamu R, Ribak CE, Friedman LK. Suppression of hippocampal neurogenesis is associated with developmental stage, number of perinatal seizure episodes, and glucocorticosteroid level. Exp Neurol 2003;184:196-213.
29 Coburn-Litvak PS, Pothakos K, Tata DA, McCloskey DP, Anderson BJ. Chronic administration of corticosterone impairs spatial reference memory before spatial working memory in rats. Neurobiol Learn Mem 2003;80:11-23.
30 Woodson JC, Macintosh D, Fleshner M, Diamond DM. Emotion-induced amnesia in rats: working memory-specific impairment, corticosterone-memory correlation, and fear versus arousal effects on memory. Learn Mem 2003;10:326-336.
31 Wong EY, Herbert J. Raised circulating corticosterone inhibits neuronal differentiation of progenitor cells in the adult hippocampus. Neuroscience 2006;137:83-92.
32 Shors TJ, Miesegaes G, Beylin A, Zhao M, Rydel T, Gould E. Neurogenesis in the adult is involved in the formation of trace memories. Nature 2001;410:372-376.
33 Watanabe Y, Gould E, McEwen BS. Stress induces atrophy of apical dendrites of hippocampal CA3 pyramidal neurons. Brain Res 1992;588:341-345.
34 Magariños AM, McEwen BS. Stress-induced atrophy of apical dendrites of hippocampal CA3c neurons: comparison of stressors. Neuroscience 1995;69:83-88.
Received August 24, 2006 Accepted after revision September 9, 2006