|
Pathologic changes in pulmonary arteries of infants with complex congenital heart defect with diminished pulmonary blood flow but without aortopulmonary collateral arteries
Xiao-Tong Zhang, Ying-Long Liu, Ying-Mao Ruan, Cun-Tao Yu, Lei Liu
Beijing, China
Author Affiliations: Pediatric Cardiac Center, Cardiovascular Institute and Fu Wai Hospital, CAMS and PUMC, Beijing 100037, China (Zhang XT, Liu YL, Ruan YM, Yu CT, Liu L); Zhang XT works in Tianjin Children's Hospital.
Corresponding Author: Ying-Long Liu, MD, Pediatric Cardiac Center, Cardiovascular Institute and Fu Wai Hospital, CAMS and PUMC, Beijing 100037, China (Tel: 86-10-88398188; Fax: 86-10-68332747; Email: liuyinglong@vip.sina.com)
Background: To evaluate the development of small pulmonary arteries of infants with complex congenital heart defect (CHD) with diminished pulmonary blood flow but without aortopulmonary collateral arteries, we compared the imaging data including diameter of the central pulmonary artery, McGoon ratio and pulmonary artery index (PAI), which were obtained by echocardiography and cardioangiography before operation.
Methods: Biopsy specimens were obtained from 46 infants suffering from complex CHD with diminished pulmonary blood flow but without aortopulmonary collateral arteries from January 2002 to September 2004. The infants were at their age of 6-36 months. They had undergone echocardiography and cardioangiography examinations. Autopsy specimens were obtained from 5 infants, aged 4-18 months, who died of non-cardiovascular diseases and served as normal controls. The tissue was fixed with buffered formalin, and routinely prepared by impregnated in wax. Tissue sections were stained with hematoxylin-eosin, Weigert's elastic stain counter-stained with van Gieson. Three parameters including percentage of media thickness (MT%), percentage of media section area (MS%) and numbers of small arterial per square centimeter (APSC) were obtained by morphologic quantitative analysis using a computer image processor. The diameters of the left pulmonary artery (LPA) and the right pulmonary artery (RPA) were measured by echocardiography and cardioangiography. The McGoon ratio and PAI were calculated according to the results of cardioangiography.
Results: There was no significant difference in MT% and MS% between the normal control group and the experimental group. The mean value was 11.44% and 18.34% in the normal control group. The mean value of APSC was significantly different between the two groups (408.58/cm2 and 217.15/cm2 respectively). The diameters of the main pulmonary artery (MPA), LPA and RPA measured echocardiographically were not correlated with pathological data (P>0.05). The diameters of the LPA and MPA (r=0.61, P=0.01), of the RPA and MPA (r=0.48, P=0.01), and of the RPA and LPA (r=0.57, P=0.01) were positively correlated. But the diameters of the LPA, RPA and MPA were not correlated with pathological parameters. The diameters of the LPA and RPA were positively correlated (r=0.378, P=0.015) as shown by cardioangiography. The McGoon ratio and PAI calculated by cardioangiography were correlated with APSC (r=0.51, P=0.001 and r=0.448, P=0.004).
Conclusion: The developing extent of small pulmonary arteries is correlated with the central pulmonary artery of infants suffering from complex CHD with diminished pulmonary blood flow but without aortopulmonary collateral arteries.
Key words: congenital heart defect; pulmonary artery; oligemia; cardioangiography; infant
World J Pediatr 2007;3(2):140-145
Introduction
The decrease of pulmonary blood flow can result in hypoplasia of the central pulmonary artery and its branches. The optimal therapeutic program lies in intracardiac abnormality and the development of distal small pulmonary arteries. The criteria which have been used to assess the growth of the pulmonary artery (PA) include simple measurement of PA size,[1] the ratio of the pulmonary artery to the diameter of the ascending[2] or more commonly the descending aorta,[3-7] McGoon ratio,[8] PA size indexed to body surface area (PAI, Nakata index),[9] and pulmonary venous index (PVI).[10] Several investigators have suggested that the diameter of the proximal left pulmonary artery (LPA), which is smaller than that of its first branch, does not always reflect the size and growth degree of peripheral pulmonary arteries, even if there is no stenosis of the proximal LPA.[11] It was found that the central pulmonary arteries grew by outgrowth from the existing vessels, a process of angiogenesis, but peripheral arteries appeared by vasculogenesis in the mesenchyme and joined with the central arteries in normal development of the pulmonary arteries.[12] There is no report about the association of the developmental degree of central pulmonary arteries and peripheral arteries with decreased pulmonary blood flow.
Although the developmental degree of pulmonary arteries can be evaluated by imaging methods, there is no criterion for accessing the development of small pulmonary arteries. The aim of the present study was to analyze the structure of small pulmonary arteries of complex congenital heart defect (CHD) with diminished pulmonary blood flow but without aortopulmonary collateral arteries in infants by quantitative morphometric techniques. The imaging data compared included the diameter of the central pulmonary artery, McGoon ratio, and PAI, which were obtained by echocardiography and cardioangiography before operation.
Methods
Patients
Between January 2002 and September 2004, we treated 46 pediatric patients with complex CHD with diminished pulmonary blood flow but without aortopulmonary collateral arteries. Among them, 26 were males and 20 females, aged 6-36 months (median age 23.42 months). The confluents of central pulmonary arteries existed. Twenty-three patients had patent ductus arteriosus (PDA) (Table 1). This study was approved by the institutional ethics committee.
Autopsy specimens were obtained from 5 infants as normal control group, who were aged 4-18 months (median age 9.8 months) and died of non-cardiovascular diseases. Patients with the following conditions were excluded: lung diseases (pneumonia, pulmonary hemorrhage, pulmonary collapse, respiratory distress syndrome, amniotic fluid inhalation, or pulmonary hypoplasia, etc), and congenital cardiovascular malformations. Infants who are premature, immature or malnutritioned were also excluded (Table 2).
Table 1. Data of the experimental group
Diagnosis |
Case number |
DORV, PS, VSD |
2 |
DORV, VSD, ASD, PS, PDA |
2 |
DORV, VSD, PS, PDA |
1 |
PA, ASD, TA, PDA |
1 |
PA, SV, TA, ASD, PDA |
1 |
PA, VSD, PDA |
12 |
SV, ASD, PS |
4 |
SV, SA, PS, PDA |
1 |
TOF |
7 |
TOF, ASD, hypoplasia of right ventricle |
1 |
SV, SA, TAPVC, PS |
1 |
SV, PDA, single coronary artery malformation |
1 |
SV, PS |
2 |
TA, VSD, PS |
2 |
TA, PS, ASD, VSD |
2 |
CAVSD, PS, TAPVC |
1 |
TGA, VSD, ASD, PS |
1 |
TGA, VSD, ASD, PS, PDA |
1 |
TGA, VSD, PDA, PS |
1 |
TOF, PDA, pFo |
2 |
ASD: atrial septal defect; DORV: double outlet right ventricle; PS: pulmonary stenosis; PDA: patent ductus arteriosus; PA: pulmonary atresia; pFo: patent oval fossa; SV: single ventricle; SA: single atrium; TA: tricuspid atresia; TOF: tetralogy of Fallot; TAPVC: total anormalous pulmonary venous connection; CAVSD: complete atrioventricular septal defect; TGA: transposition of the great arteries; VSD: ventricular septal defect.
Table 2. Data of the normal control group
Number of patients |
Diagnosis |
1 |
Congenital biliary atresia |
2 |
Acute intestinal obstruction |
3 |
Congenital nephrosis |
4 |
Congenital biliary atresia |
5 |
Polycytic kidney, polycytic liver |
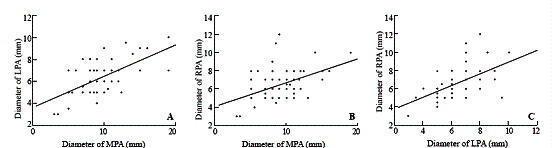
Fig. A. Relationship between the diameter of the left pulmonary artery (LPA) and the diameter of the main pulmonary artery (MPA) shown by echocardiography. The regression equation: LPA diameter (mm) = 3.57 + 0.29 ¡Á MPA diameter (mm) (r=0.61, P=0.01). Fig. B. Relationship between the diameter of the right pulmonary artery (RPA) and the diameter of the main pulmonary artery (MPA) shown by echocardiography. The regression equation: RPA diameter (mm) = 4.07 + 0.26 ¡Á MPA diameter (mm) (r=0.48, P=0.01). Fig. C. Relationship between the diameter of the right pulmonary artery (RPA) and the diameter of the left pulmonary artery (LPA) shown by echocardiography. The regression equation: RPA diameter (mm) = 2.46 + 0.65 ¡Á LPA diameter (mm) (r=0.57, P=0.01).
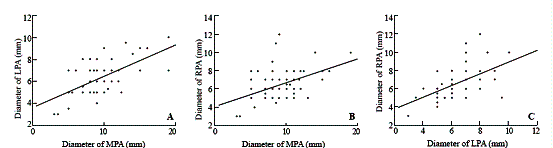
Fig. D. Relationship between the diameter of the left pulmonary artery (LPA) and that of the right pulmonary artery (RPA) shown by cardioangiography. The regression equation: LPA diameter (mm) = 3.992 + 0.352 ¡Á RPA diameter (mm) (P=0.015, r=0.378). Fig. E. Relationship between the numbers of small arteries per square centimeter (APSC) and McGoon ratio. The regression equation: APSC (/cm2) = 133.995 + 82.212 ¡Á McGoon ratio (P=0.001, r=0.51). Fig. F. Relationship between the numbers of small arteries per square centimeter (APSC) and the pulmonary artery index (PAI). The regression equation: APSC (/cm2) = 151.811 + 0.691 ¡Á PAI (mm2/m2) (P=0.004, r=0.448)
Preparation of specimens
Informed consent was obtained from the parents of patients before operation. Biopsy specimens were obtained before closure of sternotomy incision. The lung was inflated and supported by airway pressure at 2 kPa to 3 kPa. Biopsy specimens were taken from the right lower pulmonary lobe. The optimal size of the tissue was comparable to the thumb nail of the patient. Clamping of specimens should be avoided.
The autopsy lung was inflated with buffer formalin introduced through the bronchial tree until the pleural surface was intensified, and fixed for at least one week. Six blocks of tissue were taken from lung lobes of each patient (approximately 1.5 cm ¡Á 1.5 cm ¡Á 1.5 cm).
The autopsy and biopsy tissues were fixed with buffered formalin, routinely prepared by impregnated in wax. Tissue sections were stained with hematoxylin-eosin, and Weigert's elastic stain counterstained with van Gieson.
Microscopic analysis
On each section, three parameters including percentage of media thickness (MT%), percentage of media section area (MS%) and numbers of small arterial per square centimeter (APSC) were obtained by morphologic quantitative analysis using a computer image processor.[13,14]
Twelve to fifteen small pulmonary arteries were selected. The diameters of internal elastic laminae (D1) and external elastic laminae (D2) were measured under the light microscope (200 to 400 magnification). According to the formula, MT% = (2 ¡Á MT/D2) ¡Á 100% and MS% = (MS/area of small pulmonary artery section) ¡Á 100%, MT% and MS% calculated respectively reflected the wall thickness of small pulmonary arteries.
The numbers of small pulmonary arteries (N) were counted under the light microscope (200 magnification). The total area of tissue was measured on each slide. The numbers of small arteries per square centimeter (APSC) could be obtained according to the formula: APSC (/cm2) = (N/total area of tissue) ¡Á 100.
Measurement of McGoon ratio and PAI
The pulmonary valves, main pulmonary artery (MPA), LPA, and right pulmonary artery (RPA) were shown by echocardiography on the long axis cross-section at the left edge of the sternum. The diameter of the MPA was measured at the upper attachment of the pulmonary valves, and the diameters of the LPA and RPA were measured on the junction of the LPA and RPA.
All patients were reviewed on an anteroposterior cardioangiography by measuring the diameters of both right and left pulmonary arteries immediately proximal to the origin of the first lobar branches. The maximal and minimal diameters were averaged to obtain the mean diameter. The diameter of the descending aorta (DAo) near the diaphragm was measured at the same time.
McGoon ratio and PAI were calculated according to the following formula introduced by McGoon[8] and Nakata[9]:
McGoon ratio = (LPA diameter + RPA diameter) / DAo diameter; and PAI (mm2/m2) = (LPA area + RPA area) / BSA.
Cross-sectional area (mm2) = ¦Ð (diameter/2f)2. Where f is the corrective coefficient for cardioangiography magnification, BSA is body surface area in square meters, the diameter is expressed in millimeters, and the area is expressed in square millimeters.
Statistical analysis
The data were expressed as means ¡À SD with commercial statistical software package (SPSS for Windows, version 11.0; SPSS Inc, Chicardioangiograpyho, I11). Comparison of the two groups was made with Student's t test for unpaired observations. Regression and correlation analysis were made of imaging data and pathological data. P<0.05 was considered statistical significant.
Results
There was no significant difference in MT% and MS% between the normal control group and experimental group. The mean value was 11.44% and 18.34% in the normal group. MT% and MS% ranged from 5.26% to 25.50% (mean 12.07%) and 8.52% to 39.79% (mean 22.40%) respectively. The mean value of APSC was significantly different between the two groups (408.58/cm2 and 217.15/cm2 respectively). The experimental group had a lower value (Table 3).The diameters of the MPA, LPA and RPA, McGoon ratio, and PAI were measured by echocardiography and cardioangiography (Table 4).
Table 3. Pathological data of the two groups (means ¡À SD)
Group |
MT% |
MS% |
APSC (/cm2) |
Normal (n=5) |
11.44¡À4.46 |
18.34¡À5.51 |
408.58¡À78.81 |
Experimental (n=46) |
12.07¡À5.89 |
22.40¡À9.30 |
217.15¡À25.74* |
MT%: percentage of media thickness; MS%: percentage of media section area; APSC: numbers of small arterial per square centimeter. *: P<0.01.
Table 4. Imaging data of the experimental group (means ¡À SD)
|
MPA (mm) |
LPA (mm) |
RPA (mm) |
McGoon ratio |
PAI (mm2/m2) |
UCG (n=46) |
9.55¡À2.89 |
6.33¡À1.10 |
6.19¡À1.63 |
¨C |
¨C |
CAG (n=46) |
¨C |
7.15¡À1.68 |
8.52¡À2.82 |
1.69¡À0.63 |
166.42¡À49.66 |
MPA: main pulmonary artery; LPA: left pulmonary artery; RPA: right pulmonary artery; PAI: pulmonary artery index.
The diameters of the MPA, LPA and RPA were not correlated with the pathological data (P>0.05) measured by echocardiography. The diameters of the LPA, RPA and MPA were positively correlated with each other. Echocardiography showed that LPA diameter (mm) = 3.57 + 0.29 ¡Á MPA diameter (mm) (r=0.61, P=0.01) and RPA diameter (mm) = 4.07 + 0.26 ¡Á MPA diameter (mm) (r=0.48, P=0.01), respectively. The diameters of the LPA and RPA were also positively correlated. The regression equation was RPA diameter (mm) = 2.46 + 0.65 ¡Á LPA diameter (mm) (r=0.57, P=0.01) (Figs. A-C). The diameters of the LPA, RPA and MPA were not correlated with the pathological data.
The diameters of the LPA and RPA were positively correlated (r=0.378, P=0.015) as shown by cardioangiography. The regression equation was LPA diameter (mm) = 3.992 + 0.352 ¡Á RPA diameter (mm). McGoon ratio and PAI were calculated by cardioangiography. They were positively correlated with APSC (r=0.51, P=0.001 and r=0.448, P=0.004). The regression equation was APSC (/cm2) = 133.995 + 82.212 ¡Á McGoon ratio and APSC (/cm2) = 151.811 + 0.691 ¡Á PAI (mm2/m2) (Figs. D-F).
Discussion
The development of pulmonary artery bed was strongly flow-dependent.[15] In a consecutive autopsy series of 12 patients with tetralogy of Fallot and 5 patients with pulmonary atresia, muscle was found within the acinus and the wall thickness of muscular arteries was normal or minimally increased.[16] Pulmonary blood flow decreased for a long duration led to atrophy of pulmonary vascular smooth muscle along with age. The muscularity of peripheral pulmonary arteries increased and the media were thicker than the normal if pulmonary blood flow increased during pulmonary atresia with ventricular septal defect or in major aortopulmonary collateral arteries (MAPCAs).[17] In our study, although MAPCAs and the increasing muscularity of peripheral pulmonary arteries were not found under the light microscope, quantitative morphometric analysis showed a wide range of MT% and MS% and some even higher than normal. This suggests that blood filling in small pulmonary arteries and thinning of neonatal media may be affected because of the decrease of pulmonary blood flow. The use of additional pathway is more important than the dilation of existing vessels for the increasing volume of the normal lung. The normal pulmonary blood flow will stimulate the proliferation of peripheral pulmonary arteries. APSC of each patient was lower than that of the normal control group in our study, proving that the proliferation of peripheral pulmonary arteries was affected by the decreasing pulmonary blood flow. It is a common characteristic of each patient that APSC decreases in such a pathological group. It reflects the degree of hypoplasia of small pulmonary arteries.
M-mode echocardiography was used to assess PA,[18] but two-dimensional echocardiography is used at present. Although the MPA can be iden-tified echocardiographically, identification of branch, especially the LPA, is not satisfactory.[19] Echocardio-graphy can not adequately visualize the right and left branches of pulmonary arteries as well as blood flow mapping because the selection of measurement layer is affected by subjective factors.[20] Hiraishi and colleagues[11] measured the internal diameters of the MPA branches at three points along the RPA (the proximal, mid, and distal portions) and at the proximal and distal portions of the LPA in systole by both two-dimensional echocardiography and cardioangiography. The diameter of stenosis in the PA branch was also measured. These values determined by two-dimensional echocardiography were correlated well with those obtained by cardioangiography. Although two-dimensional echocardiography was well comparable to cardioangiography in diagnosing PA size in infants, the accuracy decreased because of the longer distance between the detector and measurement places, which increased measurement error in older patients.[21] In our study, only infants were included in the groups. The diameters of the MPA, LPA and RPA were determined by echocardiography selecting perfect acoustic window to measure the most inner diameter. They were correlated with each other, but not with APSC. We conclude that the MPA, LPA and RPA values detected by echocardiography do not reflect the development of peripheral pulmonary arteries accurately in infants. Echocardiography can be used for preliminary diagnosis of the developmental degree of PA in infants.
Cardioangiography is thought to be a gold standard for the diagnosis of complex CHD. It is used to determine hemodynamics and physiological conditions because of clear great vessels and accurate measurement. PAI and McGoon ratio obtained by cardioangiography are used to evaluate operation outcome and prognosis. Thus PAI and McGoon ratio are believed to reflect the physiological properties of the pulmonary vascular bed, and they are consistent with postoperative hemodynamics.[9] But PAI and McGoon ratio do not always reflect the size and development of peripheral pulmonary arteries.[22] Hofbeck and colleagues[22] reviewed their experience with total cavopulmonary anastomosis which was performed in 31 patients and concluded that poor outcome could not be predicted based on assessment of the PA size (expressed as the McGoon-ratio or the Nakata-index) alone. Reddy and colleagues[23] found that true pulmonary arteries provided vascular supply to up to 9 lung segments (median 5 segments) in pulmonary atresia with ventricular septal defect and MAPCAs. The collaterals provided vascular supply to a median of 15 lung segments per patient (range 11 to 20). The median PAI of true pulmonary arteries was 50.0 (range 0 to 103.13). The patients with aortopulmonary collaterals are excluded in our study. The measured values by cardioangiography are smaller than native values owing to hypoplasia of pulmonary arteries and softness of the arterial wall, which lead to insufficient pulmonary blood flow. Although McGoon ratio and PAI are affected by the reasons sometimes, the regression equations that McGoon ratio and PAI are correlated positively with APSC suggest that the diameters of the LPA and RPA, McGoon ratio, and PAI can reflect the growth of peripheral pulmonary arteries, as seen in our study. This finding is consistent with the relationship between PAI and peripheral pulmonary arteries.[24] Thus PAI indicates the state of the pulmonary vascular bed, especially when the small pulmonary arteries develop in proportion to the central pulmonary artery. The variation of body surface area is small in infants. It is believed that McGoon ratio is correlated with APSC. Both McGoon ratio and PAI are reliable in the assessment of the degree of development of small pulmonary arteries in infants.
There is a good relationship between the develop-mental degree of small pulmonary arteries and that of central pulmonary arteries in infants with complex CHD with diminished pulmonary blood flow but without aortopulmonary collateral arteries. To investigate the developmental degree of peripheral pulmonary arteries before operation, APSC can be calculated by regression equation, providing a morphological foundation for the therapeutic regimen.
Funding: This study was supported by a grant from the Key Science and Technologies Program of National Tenth-Five-Year Plan (2002BA709B09).
Ethical approval: This study was approved by the institutional ethics committee.
Competing interest: None declared.
Contributors: ZXT wrote the main body of the article under the supervision of LYL. LYL is the guarantor. RYM and YCT provided advices on medical aspects. LL is the helper in the pathological analysis.
References
1 Cotrufo M, Arciprete P, Caianiello G, Fittipaldi O, de Leva F, Violini R, et al. Right pulmonary artery development after modified Blalock-Taussig shunt (MBTS) in infants with pulmonary atresia, VSD and confluent pulmonary arteries. Eur J Cardiothorac Surg 1989;3:12-15.
2 Tucker WY, Turley K, Ullyot DJ, Ebert PA. Management of symptomatic tetralogy of Fallot in the first year of life. J Thorac Cardiovasc Surg 1979;78:494-501.
3 Guyton RA, Owens JE, Waumett JD, Dooley KJ, Hatcher CR Jr, Williams WH. The Blalock-Taussig shunt. Low risk, effective palliation, and pulmonary artery growth. J Thorac Cardiovasc Surg 1983;85:917-922.
4 Gale AW, Arciniegas E, Green EW, Blackstone EH, Kirklin JW. Growth of the pulmonary anulus and pulmonary arteries after the Blalock-Taussig shunt. J Thorac Cardiovasc Surg 1979;77:459-465.
5 Piehler JM, Danielson GK, McGoon DC, Wallace RB, Fulton RE, Mair DD. Management of pulmonary atresia with ventricular septal defect and hypoplastic pulmonary arteries by right ventricular outflow construction. J Thorac Cardiovasc Surg 1980;80:552-567.
6 Ilbawi MN, Grieco J, De Leon SY, Idriss FS, Muster AJ, Berry TE, et al. Modified Blalock-Taussig shunt in newborn infants. J Thorac Cardiovasc Surg 1984;88:770-775.
7 McKay R, de Leval MR, Rees P, Taylor JF, Macartney FJ, Stark J. Postoperative angiographic assessment of modified Blalock-Taussig shunts using expanded polytetrafluoroethylene (Gore-Tex). Ann Thorac Surg 1980;30:137-145.
8 McGoon DC, Baird DK, Davis GD. Surgical management of large bronchial collateral arteries with pulmonary stenosis or atresia. Circulation 1975;52:109-118.
9 Nakata S, Imai Y, Takanashi Y, Kurosawa H, Tezuka K, Nakazawa M, et al. A new method for the quantitative standardization of cross-sectional areas of the pulmonary arteries in congenital heart diseases with decreased pulmonary blood flow. J Thorac Cardiovasc Surg 1984;88:610-619.
10 Kawahira Y, Kishimoto H, Kawata H, Ikawa S, Ueda H, Nakajima T, et al. New indicator for the Fontan operation: diameters of the pulmonary veins in patients with univentricular heart. J Card Surg 1999;14:259-265.
11 Hiraishi S, Misawa H, Hirota H, Agata Y, Horiguchi Y, Fujino N, et al. Noninvasive quantitative evaluation of the morphology of the major pulmonary artery branches in cyanotic congenital heart disease. Angiocardiographic and echocardiographic correlative study. Circulation 1994;89: 1306-1316.
12 deMello DE, Sawyer D, Galvin N, Reid LM. Early fetal development of lung vasculature. Am J Respir Cell Mol Biol 1997;16:568-581.
13 Dunnil MS. Measurement in pathology. Hum Pathol 1975;6: 138-139.
14 Zhang XT, Ruan YM, Liu YL, Yu CT. Quantitative structural study of pulmonary artery in patients with pulmonary atresia with ventricular septal defect. Zhongguo Yi Xue Ke Xue Yuan Xue Bao 2004;26:241-246.
15 Borowski A, Reinhardt H, Schickendantz S, Korb H. Pulmonary artery growth after systemic-to-pulmonary shunt in children with a univentricular heart and a hypoplastic pulmonary artery bed. Implications for Fontan surgery. Jpn Heart J 1998;39:671-680.
16 Rabinovitch M, Herrera-deLeon V, Castaneda AR, Reid L. Growth and development of the pulmonary vascular bed in patients with tetralogy of Fallot with or without pulmonary atresia. Circulation 1981;64:1234-1249.
17 Haworth SG, Macartney FJ. Growth and development of pulmonary circulation in pulmonary atresia with ventricular septal defect and major aortopulmonary collateral arteries. Br Heart J 1980;44:14-24.
18 Goldberg BB. Suprasternal ultrasonography. JAMA 1971;215:245-250.
19 Gutgesell HP, Huhta JC, Cohen MH, Latson LA. Two-dimensional echocardiographic assessment of pulmonary artery and aortic arch anatomy in cyanotic infants. J Am Coll Cardiol 1984;4:1242-1246.
20 Greenberg SB, Crisci KL, Koenig P, Robinson B, Anisman P, Russo P. Magnetic resonance imaging compared with echocardiography in the evaluation of pulmonary artery abnormalities in children with tetralogy of Fallot following palliative and corrective surgery. Peditr Radiol 1997;27:932-935.
21 Fogel MA, Donofrio MT, Ramaciotti C, Hubbard AM, Weinberg PM. Magnetic resonance and echocardiographic imaging of pulmonary artery size throughout stages of Fontan reconstruction. Circulation 1994;90:2927-2936.
22 Hofbeck M, Singer H, Scharf J, Wild F, Ries M, Mahmoud O, et al. Total cavopulmonary anastomosis: selection criteria related to postoperative results. Thorac Cardiovasc Surg 1993;41:28-33.
23 Reddy VM, Liddicoat JR, Hanley FL. Midline one-stage complete unifocalization and repair of pulmonary atresia with ventricular septal defect and major aortopulmonary collaterals. J Thorac Cardiovasc Surg 1995;109:832-845.
24 Senzaki H, Kato H, Akagi M, Hishi T, Yanagisawa M. Relationship between the pulmonary artery index and physiological properties of the pulmonary vascular bed. Jpn Circ J 1996;60:334-340.
Received November 20, 2006; Accepted after revision March 29, 2007
|